Omic.ly Weekly 71
April 14, 2025
Hey There!
Thanks for spending part of your week with Omic.ly!
This Week's Headlines
1) How does the brain control perception of everything around us?
2) The proteasome and lysosome decide what proteins live or die
3) Jelly fish proteins started brightening up microscopy in 1994
Here's what you missed in this week's Premium Edition:
HOT TAKE: "De-extincted" Wolves, A Discontinued Sequencer, and more layoffs for PacBio
Deep brain structures play a key role in filtering out what we perceive
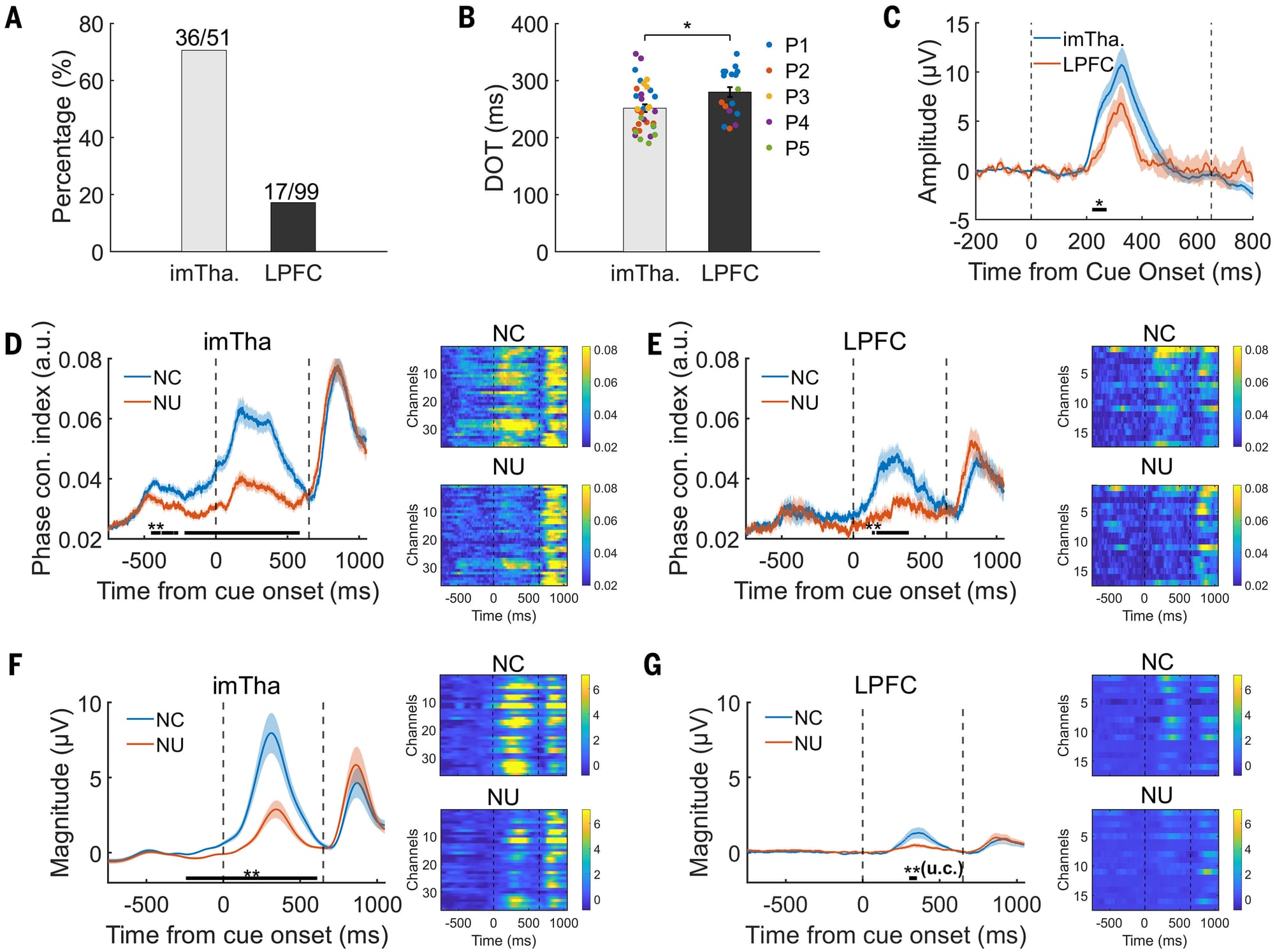
At any given moment, we're surrounded by thousands of stimuli!
Even now, while you're reading this, you're probably sitting down somewhere focused on the words that are passing before your eyes.
But what about all of the things around you?
Maybe you're in a coffee shop or on a train, how does your brain decide to let you focus on these words and not the people walking by, the sounds you're hearing in the background, or the icons flashing in the notification bar on your phone or computer screen?!
Awareness of the world around you (either your physical environment or the thoughts in your head) is referred to as consciousness.
But you can't be conscious of everything that's happening in your brain or your surroundings, so how does the brain decide what you perceive at any given moment?
This has been an unanswered question for a very long time, but studies of the brain have shown that the prefrontal cortex (PFC) and especially the lateral portion (LPFC) are involved in consciousness perception.
But much of what we know about consciousness perception, particularly in humans, is limited to studying the outer structures of the brain.
This is because studying the inner structures requires highly invasive surgery; however, recent studies of the brains of mice have shown that while consciousness perception does involve the LPFC, other, deeper brain structures like the thalamus might play a significant role in filtering what the brain focuses on.
Brain modeling studies corroborate this 'thalamic' hypothesis that it plays an important role in gating consciousness perception before passing those signals to the outer structures of the brain.
But the brains of mice don't always behave the same way that our brains do!
Fortunately, researchers at Beijing Normal University realized they had access to the perfect test population for studying consciousness perception in humans.
They had 5 patients enrolled in a headache study who had stereoelectroencephalography (sEEG) electrodes implanted in their PFC and thalamus.
These electrodes can be used to stimulate the brain or to measure brain activity in the structures where they've been implanted.
And since these participants were otherwise healthy individuals, they were ideal test subjects for a consciousness study.
They were subjected to a novel visual perception test and their brain activity was measured to see how it changed when they indicated they perceived a visual cue vs when they didn't perceive that cue.
The perception results can be seen in the figure above which shows that consciousness signals were much stronger in the thalamus than in the LPFC (A) and the thalamus perceived these signals about 200ms sooner than the LPFC (B). C) shows a graph of the amplitude of the signals measured in each brain region. D,E,F, and G) break this out further by comparing conscious (NC) detection of the visual cue to non-conscious (NU) detection and you can see the signals appear sooner and stronger in NC than NU (peak between dashed lines). Dashed lines here indicate the duration of the visual cue followed by a second, much more obvious, visual cue for the participant to signal if they saw the first visual cue.
These results indicate that consciousness perception originates in the Thalamus (specifically the intralaminar and medial thalamic nuclei) and connects to the prefrontal cortex.
The results also corroborate those previous findings in mice!
This work is important because it provides a new understanding of how deep brain structures like the thalamus contribute to consciousness perception.
###
Fang Z, et al. 2025. Human high-order thalamic nuclei gate conscious perception through the thalamofrontal loop. Science. DOI: 10.1126/science.adr3675
The importance of taking out the trash: An overview of cellular protein degradation pathways.
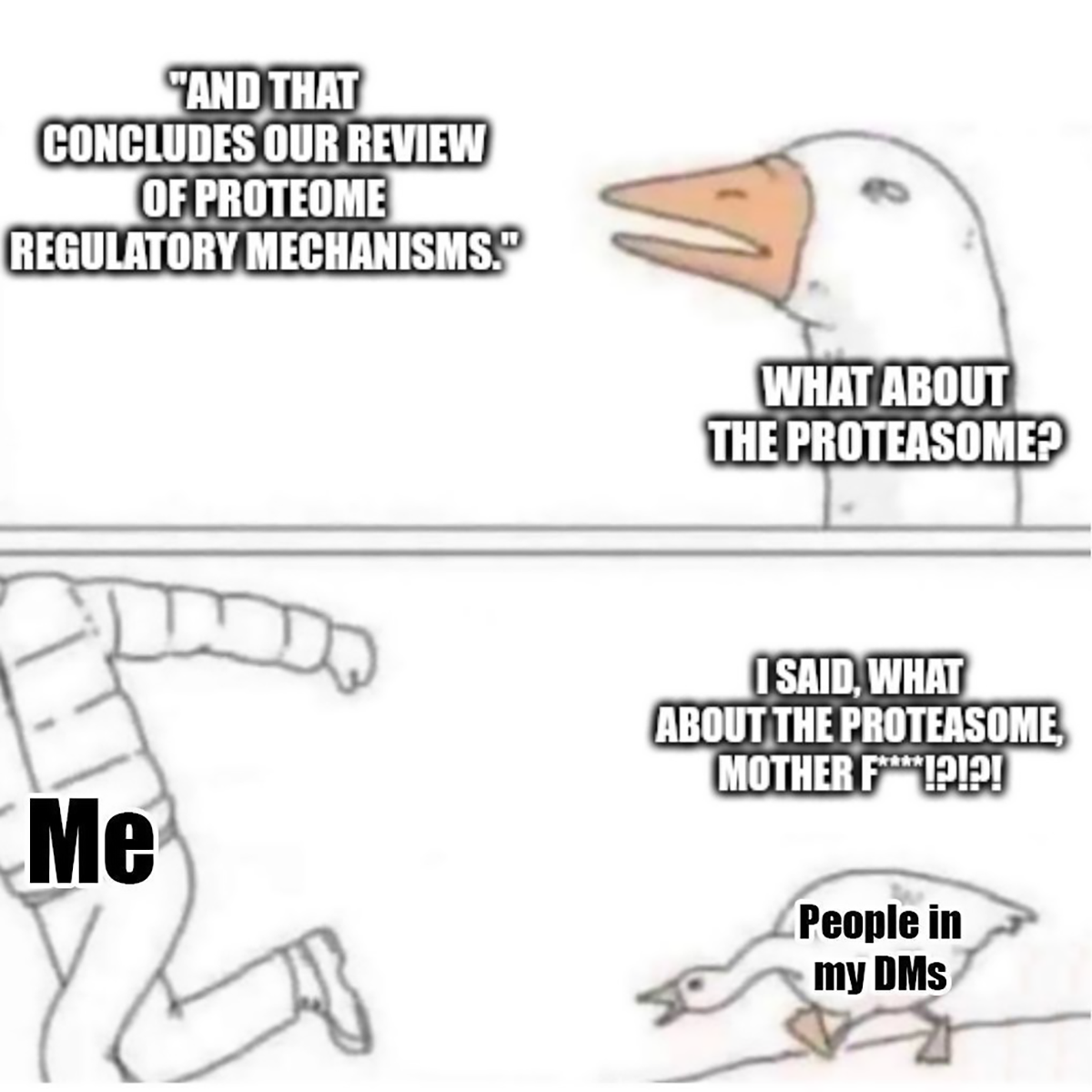
This might not be obvious, but cells generate a lot of garbage.
Like anything, parts of cells get damaged or worn out and eventually need to be replaced.
This is true of small proteins all of the way up to mitochondria or other large organelles.
Some cells, like those in our immune system, even EAT THINGS like bacteria or viruses which are really just juicy bags of protein that need to be degraded!
But it’s not only broken proteins or microbes that get disposed of.
During development, cell division, or even throughout the day, a cell’s needs change.
This means that the proteins that are present also need to change!
This is especially true when cells ‘differentiate’ into all of the different cells that make up our organs and tissues.
And, if you remember, YOU are the product of one sperm and one egg that divided into trillions of different cells!
To get from a fertilized egg to YOU, changes in protein content within all of those cells needed to be made.
And the changing protein needs of cells are, in part, regulated by protein degradation pathways!
There are two major ways to chop up proteins in a cell:
The Proteasome
These are like protein wood chippers. They’re used to help with the rapid proteolysis (big word for chopping) of useless, misfolded, damaged, or no longer needed proteins!
The proteasome is itself a protein complex that recognizes a specific tag on proteins marked for destruction and dices them up into ~7 amino acid chunks.
Those chunks can then be further degraded and reused to create new proteins!
The tag that the proteasome recognizes is a 76-amino acid protein called ubiquitin.
And this tag is added by a grim reaper-like protein called ubiquitin ligase.
Once a chain of at least 4 ubiquitins is added to a protein, it is recognized by the proteasome and gobbled up.
The Lysosome
If the proteasome is a wood chipper, then the lysosome is like an industrialized garbage dump.
The lysosome is a specialized membrane enclosed organelle (kind of like a bathroom inside a house) where bulk protein degradation activities take place.
They’re filled with proteases (small protein chomping enzymes) and have a highly acidic environment to aid in the breakdown of whatever is unfortunate enough to end up in there.
The lysosome is used for digesting nutrients that have been brought in from outside the cell, degrading proteins that have hung around for too long, disassembling and recycling major cellular organelles like mitochondria (autophagy), or bulk destroying proteins during times of stress.
And it's probably not surprising that problems with both the proteasome and the lysosome are implicated in many diseases.
Because, proteostasis, or the maintenance of the proteome through the creation and destruction of proteins, is key for normal cellular function!
In 1994, an obscure jellyfish protein was stuck into a worm. It has enlightened biology and medicine ever since.
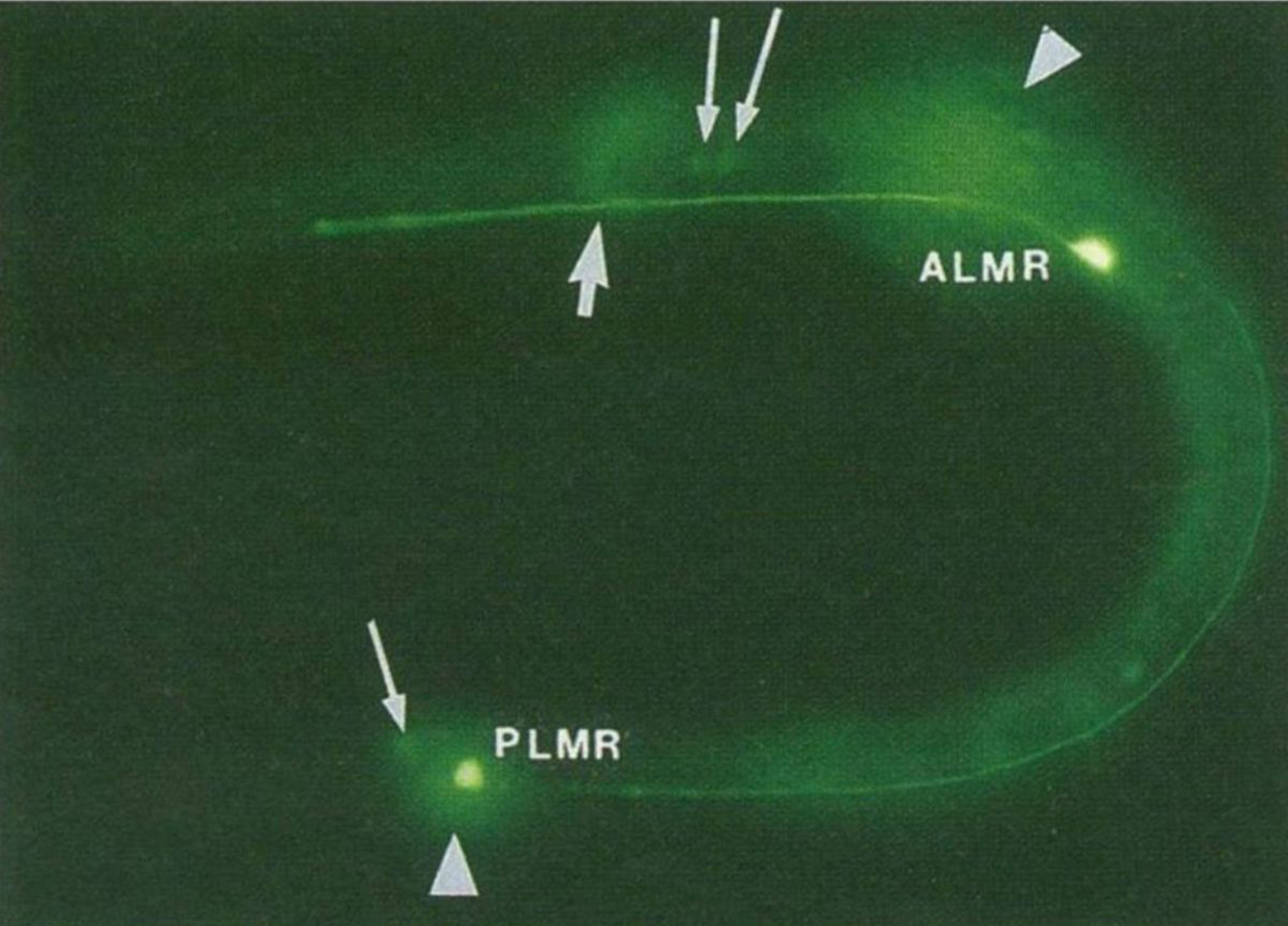
But before we get to the genetically engineered worm, we first have to talk about the discovery of that jellyfish protein in 1960.
Aequorea aequorea, the jellyfish species in question, might seem like an odd place to start, but jellyfish are bioluminescent.
Osamu Shimomura was interested in studying the chemistry and physics of how these animals were able to produce their blue-green glow.
Up until that point, it was known that most bioluminescent creatures, like fireflies, do so with an enzymatic reaction between luciferin and luciferase.
But Shimomura discovered something different!
And in 1962 he isolated aequorin, a calcium binding protein that glows blue, along with another protein that had a bright green color under UV light.
Over the next decade, Shimomura dissected how aequorin and the green protein worked together to produce the characteristic glow of these jellyfish.
As it turns out, the blue calcium binding protein excites the green protein!
And in 1974 he published a paper describing what we now know of as green fluorescent protein (GFP).
Although Shimomura discovered the protein, he didn't see any further use for it and so focused his research elsewhere.
However, a few years later, this work was picked back up by Doug Prasher.
Unfortunately, getting a hold of enough protein to do anything useful with it was challenging because it had to be harvested from animals.
Prasher got the bright idea that he could use a new technique called cloning to make a bunch of this protein without having to collect and dissect a ton (literally) of jellyfish.
He was the first to sequence the GFP gene, but he couldn't get the cloned protein to light up, and so he gave up after publishing a paper about cloning the gene.
Soon after, Prasher was contacted by two labs who saw his paper and were interested in using GFP as a molecular tracer for imaging.
Martin Chalfie and his rotation student, Ghia Euskirchen, got the first shot and found that the original clone didn't work because it contained a couple of extra amino acids that prevented the protein from properly folding.
The figure above is the result of putting that now functioning sequence into C. elegans, a nematode worm.
It was expressed using an ALM/PLM neuron specific promoter (bright green dots).
But the story doesn't end there!
The second lab to get Prasher's GFP clone was Roger Tsien's.
Tsien was a biochemist and had visions for GFP beyond just green ones.
His lab created much of the fluorescent protein rainbow that we have available today.
Shimomura, Chalfie, and Tsien shared the Nobel Prize for this work in 2008.
And fluorescent proteins are now a ubiquitous molecular tool that color everything from cell cultures to aquarium fish!
###
Chalfie M, et al. 1994. Green fluorescent protein as a marker for gene expression. Science. DOI:10.1126/science.8303295
Were you forwarded this newsletter?
LOVE IT.
If you liked what you read, consider signing up for your own subscription here: